Life adapted to precariousness
The ecology of drylands
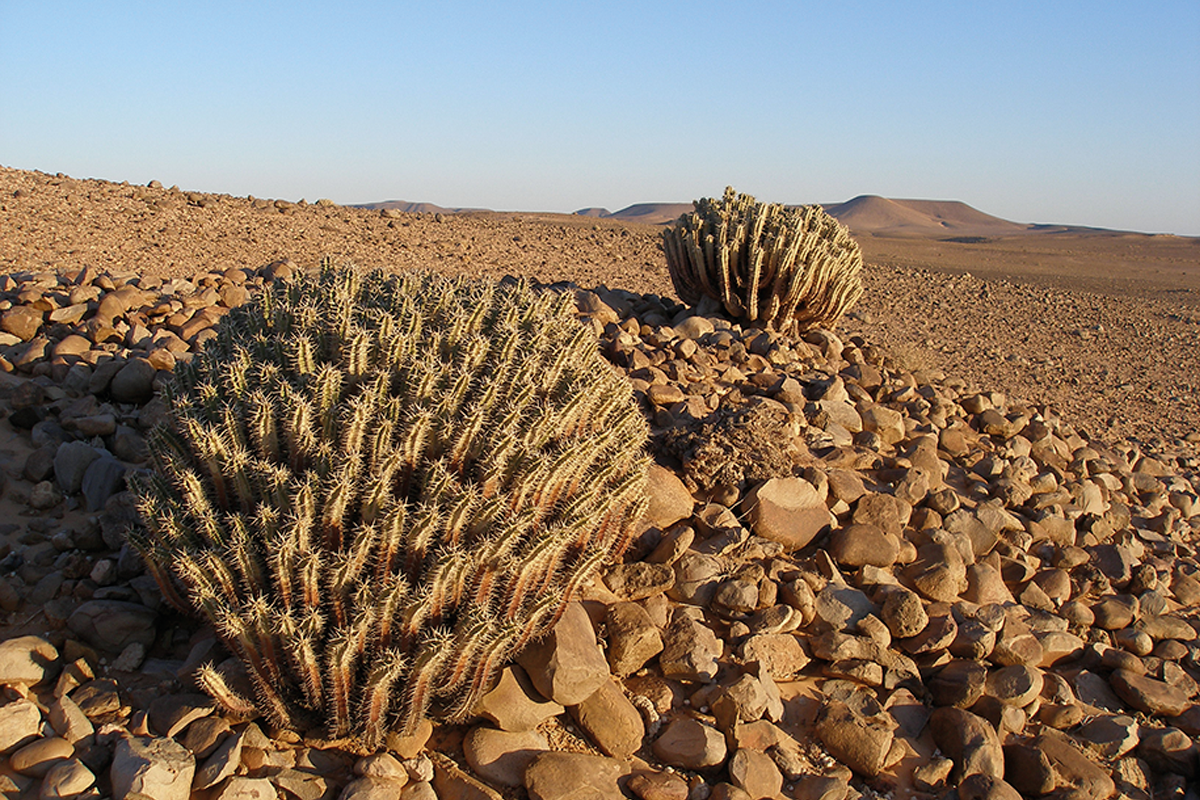
Drylands occupy approximately 40 % of the Earth’s surface. Their peculiar hydrological system, with water as the main limiting factor, together with other characteristics such as the variability of rainfall and their ecological heterogeneity, make these regions one of the main and most relevant sets of biomes on the planet. Beyond their stereotypical conception as places with low economic and ecological profiles, these territories have enormous biodiversity and support 40 % of the world’s population. Global warming is increasing atmospheric aridity, meaning that the strategies developed over millennia by the inhabitants of these areas represent a model we can learn from. Preserving these places is essential to combat climate change, and to do so we must have an in-depth understanding of their structure and functioning.
Keywords: aridity, droughts, biodiversity, adaptations, desertification.
The identity, distribution, and importance of drylands
Water is the first element that comes to mind when talking about drylands. Its scarcity formally defines what is arid and what is not. We currently use the aridity index (AI) – the ratio of annual precipitation to annual potential evapotranspiration1 (PET) – to quantify the moisture deficit of a location.2 We speak of drylands when the aridity index falls below 0.65. There are four categories of aridity (dry sub-humid, semi-arid, arid, and hyper-arid), as can be seen in Figure 1. Depending on the method3 used to calculate annual PET, the distribution and extent of drylands varies. Thus, according to the World Atlas of Desertification (WAD) (Cherlet et al., 2018), these areas occupy 37.2 % of the Earth’s surface (Figure 1), while the Intergovernmental Panel on Climate Change (IPCC) states that they extend across 46.2 % of the planet (Mirzabaev et al., 2019). Furthermore, differences in the resolution of the data used and the periods selected also result in substantial differences between the different areas estimated.
Figure 1. Distribution of the world’s drylands according to their aridity category (UNEP-WCMC, 2007). Depending on the methods used to calculate it, between 37.2 % and 46.2 % of the surface area of the planet is estimated to consist of drylands.
While scarce rains are intuitively and formally linked to drylands, the key determinant for the structure and functioning of their ecosystems is the spatial and temporal variability of rainfall, which increases with aridity (D’Odorico et al., 2013). The lack of precipitation during part of the year is another hallmark of these regions. Although droughts affect almost all climatic regions, and more than half of the Earth is susceptible to them, the presence of dry seasons is characteristic of drylands, where off-season droughts can also occur. It can be difficult to determine when a drought begins or ends, and its effects can extend over a wider geographical area than other natural hazards, such as fires or floods. This makes them one of the most significant natural disasters in terms of the number of people they directly affect (Hewitt, 1997). There are different types of droughts, depending on the segment of the hydrological cycle we are referring to. Meteorological drought refers to the lack of precipitation. Hydrological drought can be defined as a decrease in the availability of surface water and groundwater in a managed system over a given time period (with respect to average values). Finally, agricultural drought can be understood as the deficit of sufficient moisture in the root zone to meet the needs of a crop at a given location during a given season. Seasonal droughts can also result in annual droughts of indeterminate duration; for example, decades-long droughts are common in the Sahel. Hence, given the uncertainty associated with drought durations, food, water, and nutrient reserves are essential to overcome these periods of scarcity. Groundwater, seed reservoirs, the structures of succulent plants – which act as true plant cisterns (Martínez-Valderrama, 2016), or herds of animals (which, albeit starved, represent living stores of potential food, as literally indicated by the term livestock), are examples that illustrate the pulse-reserve conceptual model (Reynolds et al., 2004). This idea, which relates rainfall to pulses of primary productivity that accumulate in the form of carbon and energy, is attractive and has been widely used to explain the ecology of drylands.
Regarding the orography, drylands are often characterised by large plains, such as Patagonia in South America or the steppes of Central Asia and Mongolia. However, the presence of mountain relief is key to the water supply in these areas. On the one hand, precipitation on gradients increases with altitude. The small coastal mountain ranges in the southeast of the Iberian Peninsula, for example, can range from 200 mm on the coast to 600–800 mm on their summits. Only here is rainfall sufficient to generate runoff and recharge groundwater. On the other hand, more than 8.5 % of drylands are situated more than 1,000 metres above sea level (FAO, 2011). These mountain systems are considered the «water towers» or «castles» that store water and regulate runoff, thereby extending their influence over large and often distant territories. The Atlas Mountains in Morocco, much of the Andes, and some of the most formidable mountain ranges in Asia – such as the Hindu Kush, Pamir, and Kunlun – play a key role in the water cycle of these drylands.
The climatological characteristics of drylands, coupled with the relatively low fertility of their soils, impose significant constraints on their biota. The poor quality of dryland soils (Plaza et al., 2018) stems from water scarcity, which slows down their regeneration processes, not least because the presence of microorganisms such as microbes and fungi decreases with aridity because of their strict dependence on moisture (MEA, 2005). As a consequence of these constraints, compared to wetter ecosystems, dryland vegetation is often sparse and scattered, forming a «biphasic» mosaic (Figure 2) in which discrete patches of vegetation (mostly grasses and shrubs) are separated by a matrix of bare soil and soil biocrusts dominated by lichens, mosses, and cyanobacteria (Maestre et al., 2012). Such scarcity of vegetation, which becomes more significant as the level of aridity increases, their harsh climate and some environmental problems caused by poor management of their natural resources, have contributed to the general public’s perception of drylands as degraded and «useless» ecosystems, both in ecological and socio-economical terms.
It is indeed common to equate low productivity with degradation and to mistake desertification – as we know the processes of degradation in drylands – with the advance of deserts (Martínez-Valderrama, 2016). To put the productivity of these areas into perspective and compare them with others, the concept of rainfall use efficiency (RUE) (Le Houérou, 1984), which can be interpreted as the amount of plant biomass produced per unit of precipitation, can be useful. It is estimated that 10–20 % of drylands have desertification problems. These numbers are likely to increase significantly as a result of climate change, projected human population growth in the coming decades (especially pronounced in sub-Saharan Africa, where the population is expected to double by 2050), expansion of cropland, and unsustainable land management practices (FAO, 2021).
Despite the seemingly unstoppable advance of land degradation in drylands, these places are of paramount importance to humans for multiple reasons. First, they are home to between 2 billion (UNCCD, 2017) and 3 billion (Mirzabaev et al., 2019) people. These figures include the most vulnerable populations, given that many developing countries have extensive drylands. More than 1.3 billion people are trapped on degraded agricultural land, are exposed to climate stress, and are excluded from economic development (UNCCD, 2017). The importance of drylands is also reflected in other magnitudes: 30 % of crops originate here; they host 36 % of carbon stores and almost 30 % of forested areas; and they support 50 % of the world’s livestock and 44 % of cropland (Cherlet et al., 2018). In addition, drylands hold strategic resources, including most of the world’s oil reserves, as well as large deposits of valuable minerals such as precious metals (gold, copper, and silver), and many of the fundamental components of today’s technology (lithium, copper, and rare earth metals).
Adaptations and biodiversity: the fascinating biota of drylands
Contrary to suggestions of the collective imagination, drylands are quite biodiverse ecosystems. Their unexpected assortment of life is a consequence of the adaptations of their biota to the specific conditions of these regions. Arid ecosystems are not only highly diverse, they also provide a fascinating natural laboratory for studying the evolution and adaptation of species to extreme conditions, as well as offering ecosystem services that are essential for sustaining life. For example, they host around 20 % of the world’s major centres of plant diversity and include hotspots of plant biodiversity such as the South African Fynbos belt (Maestre et al., 2021).
Figure 2. Dryland vegetation is often sparse and scattered. The images show several examples of the landscape found in drylands around the world. (A) A savannah in Kenya. (B) A scrubland in the Patagonian Steppe, Argentina. (C) Bou-Hedma National Park, Tunisia. (D) Aleppo pine (Pinus halepensis) groves in Alicante. (E) Tabernas Desert, Almeria. (F) Atlantic Sahara. / Photos: Vicente Polo (A), Juan José Gaitán (B), Fernando Maestre (C), Fernando Maestre (D), Leo Barco (E), and Associació Harmusch (F)
In addition to their high plant diversity, which in some cases exceeds what we can find in more productive biomes, dryland ecosystems also harbour very diverse microbial and edaphic communities. This biodiversity is crucial for maintaining their functionality while simultaneously providing multiple ecosystem services, as suggested by recent studies of vascular plants and biocrusts around the world. In addition, drylands contain a wide variety of vegetation (Figure 2) and soil types. For example, a global study of 224 arid ecosystems in 16 countries identified 26 different soil types (Maestre, 2012).
Far from the conventional image of dusty, abiotic plains, it might come as a surprise to readers less familiar with these environments that more than a quarter of the world’s forested areas are found in drylands (FAO, 2019). Almost one-third of the world’s drylands are forested, amounting to 1.1 billion hectares of forest globally. These forested areas provide habitats for biodiversity, protect land from erosion, provide shade, help water penetrate soils, and contribute to soil fertility. In addition to forests or woodlands (which cover 28 % of drylands), 25 % of drylands are grasslands and 14 % are croplands. The remaining 28 % corresponds to more stereotypical drylands: barren places with little vegetation where life finds a way through incredible adaptations.
Dryland plants have developed a set of adaptations and strategies to escape, evade, resist, or withstand the climate conditions present in these places (Maestre et al., 2021). The C3 carbon fixation metabolic pathway is used in most plants; however, the C4 carbon fixation and Crassulacean acid metabolism (CAM) pathways represent drought-adapted alternatives to C3 fixation. Nonetheless, the C4 and CAM pathways sacrifice efficiency to reduce water loss (because they do not need to keep their stomata open as long). These designations allude to their biochemical mechanisms and, in essence, represent a key adaptation for their survival in hot, dry places.
Terophytes are short-lived plants that survive in seed form during unfavourable seasons. The seeds of many annual plant groups have very thick seed coats that need to be scarified by sand and gravel as they are moved by wind, or during flooding or runoff, before they will germinate. Until scarification is complete, the seeds are viable for decades, effectively becoming propagules that travel through time and space until they find the right conditions to germinate. Perennial plants, such as brittlebush (Encelia farinosa) in northern Mexico and the southwestern United States, avoid the effects of drought by losing their leaves during the harshest part of the dry season. Oher plants can also reduce transpiration via stem photosynthesis, as is the case with yellow broom (Retama sphaerocarpa) in southern Europe.
Still other plants have developed water and nutrient storage tissues above or below ground to resist drought. This is the case of succulent plants like living stones (Lithops spp.) or the emblematic saguaro (Carnegiea gigantea). Most succulents store water in mucilage or pectic gel. In addition, they have shallow and wide rooting systems that grow rapidly immediately after rainfall events and die when the soil dries out (hence their name, «rain roots»). Some evergreen trees and shrubs use their deep root systems and thick bark to withstand drought. Indeed, root depths of more than 50 metres have been recorded for species such as tamarisk (Tamarix spp.) or jujube (Ziziphus lotus). Deep roots are often coupled with extreme longevity, which helps them ensure long-term survival when seed predation is high and seedling survival is very low.
The vertical structures of cacti and the vertical leaves of several succulent taxa reduce their exposure to dangerous levels of infrared and ultraviolet light. Columnar cacti such as C. gigantea use their cuticle and epidermis to absorb ultraviolet radiation and reflect infrared radiation. The small leaves also help to avoid excessive exposure to sunlight, thus limiting heat stress. Trichomes, fine «hair-like» appendages covering leaves and stems, help to avoid heat by reducing leaf absorption by reflecting infrared radiation and also by reducing transpiration rates, thereby contributing to water conservation in plant tissues. Thorns also play a small role in reducing daytime temperatures while increasing night time temperatures in cacti, euphorbias, or acacias.
Figure 3. The distribution of land use in drylands. The type of barren landscapes with little vegetation popularly associated with these regions occupy only 28 % of their surface area. The same percentage is covered by forests or wooded areas and, of the remainder, 25 % is grassland and 14 % cropland. / Source: FAO (2017)
The morphological and physiological adaptations of animals are essentially no different from those used by plants (Martínez-Valderrama, 2016). Cooling mechanisms that prevent the temperature from exceeding critical thresholds are required in these environments. The temperature of mammals and birds are constitutionally abnormally high. This causes them to pant and sweat to promote water evaporation and cool them down as heat is extracted from their bodies during the state change from a liquid to a gas. Many animals, such as the fennec fox (Vulpes zerda) have highly developed external ears that act as heat-dissipating surfaces, with blood being cooled as it circulates through them. Some birds, such as the Namaqua sandgrouse (Pterocles namaqua), fill their plumage with water and can fly wrapped in a watery film that keeps them cool. The same insulating structures that prevent water loss are also used to mitigate overheating. For instance, air chambers formed under plumage or fur are an excellent insulator against high temperatures.
One strategy is unique to animals, because of a crucial difference with plants. They can move around, avoiding water scarcity and high temperatures. This translates into habits that tend to be nocturnal, along with daily or seasonal movements in search of resources. We can think of wild herbivores (gazelles or guanacos, etc.) or domestic herbivores (goats, sheep, or dromedaries, etc.) as the most representative of this behaviour. In addition, their predators will closely follow in their footsteps. Thus, the vagaries of rain set the whole animal kingdom in motion. Livestock transhumance and nomadism are a faithful reflection of human behaviour towards wild animals.
Drylands and the Sustainable Development Goals
Due to the pressure on drylands, their degradation accentuates their natural scarcity and variability of resources. This deterioration, which is partly due to climatic variations spurred by global warming and partly because of inadequate resource management, is known as desertification. The main challenge for safeguarding drylands and ensuring the well-being of the billions of people who live there (including Spanish citizens: let us remember that 73 % of Spain are drylands) is to reconcile economic activity with ecosystem performance. The ambitious and multifaceted Sustainable Development Goals (SDGs) set out in 2015 by the United Nations (UN, 2015) are the way to address this challenge. There are 17 goals broken down into 169 targets to be achieved by 2030.
Figure 4. The unexpected assortment of life in these areas is a result of the adaptations of their biota to the specific conditions of these regions. The picture shows typical dryland vegetation: (A) Euphorbias, (B) acacias in the Atlantic Sahara, (C) a jujube tree (Ziziphus lotus) in Almeria, and (D) candelabra cactus (Jasminocereus thouarsii) in the Galapagos Islands, Ecuador. / Photos: Harmusch Association (A), Harmusch Association (B), Emilio Guirado (C), and Fototeca CENAM (D)
The target specific to drylands is 15.3, aims to «combat desertification, restore degraded land and soil, including land affected by desertification, drought and floods, and strive to achieve a land degradation neutral world» (Lucatello & Huber-Sannwald, 2020). To achieve this, it sets out an ambitious plan: to achieve land degradation neutrality by 2030. In essence, the aim is to compensate for areas that are being degraded as a result of various human needs (e.g., cultivating land). It aims to restore these areas through regenerative agriculture or restoration schemes to achieve an overall neutral or positive balance.
In addition to SDG 15, the drylands in which some of the world’s poorest populations live, are the raison d’être for some of the other main goals. Thus, a fundamental objective is to eradicate poverty (SDG 1). Other goals promote gender equality (SDG 5), food security (SDG 2), health (SDG 3), economic prosperity (SDG 8), safe drinking water, the quality and quantity of drinking water and sanitation (SDG 6), and climate change control (SDG 13). As can easily be deduced, synergies and contradictions between such ambitious and disparate goals are common. For example, many of the desertification problems in sub-Saharan Africa can be solved by empowering women. Better education (SDG 4) translates into more further economic independence and lower birth rates. Furthermore, reducing the gender gap (SDG 5) is also key to decrease degradation rates (SDG 15). However, improving some things inevitably makes others worse. A recent study (Vanham et al., 2021) showed that reserving a minimum share of water for ecosystems improves SDG 2 («zero hunger») by providing fish for nutrition, or SDG 15 («life on land») by maintaining habitats. Nevertheless, these changes also worsen SDG 6 indicator 6.4.2 related to water stress. Specifically, the minimum water flow essential for ecosystem survival means that 32 % of the world’s population will be water-stressed for at least one month per year and 14 % will be water-stressed for at least six months per year.
Conclusions and perspectives
Due to the volatility of rainfall, nature in the drylands is highly variable and seemingly chaotic. However, behind these random fluctuations, nature flourishes in all its splendour whenever it has the opportunity. Drylands are home to some of the organisms most resilient to adverse environmental conditions on planet Earth. These include plants that can remain dormant for years that are resurrected after a fleeting shower of rain. The diverse cultures that have thrived in these territories are testimony to this. Humans have survived for millennia in these regions thanks to exquisite management of the available resources. However, austerity is an incentive to improve, and the opportunistic nature of the inhabitants of drylands manifests itself in their ability to take advantage of every opportunity. Technological developments, wet periods, and other favourable circumstances have created economic systems that often exceed the possibilities of the environment. In many cases, continuous exhaustion of drylands, whose fluctuating nature has been exacerbated by climate change, has resulted in severe degradation processes. At their worst, these changes have driven out their inhabitants and, at their best, they have made them dependent on external resources. Key aspects to maintaining the planet’s most extensive set of biomes include highlighting the inherent richness of drylands, awareness of new opportunities for development in these territories, and knowledge of how to manage forms of coexistence between economic well-being and the preservation of their ecosystems. All these factors are fundamental to safeguard the sustainability and the well-being of their inhabitants in an increasingly arid world.
Notes
1. The amount of water that could be lost through direct evaporation and transpiration from vegetation if water availability were unlimited. (Go back)
2. Different indices are available to characterise aridity. Many of them, such as Lang or Martonne’s, compare precipitation and temperature. Others, such as Meigs’s, include potential evapotranspiration (PET). The prevalence of the use of the AI results from its adoption by the United Nations (UN) Environment Programme. Following the UN Convention to Combat Desertification, most institutions and agencies (both national and international) now use the AI to designate which territories are considered drylands. Some examples of institutions that use this index include the Intergovernmental Panel on Climate Change (IPCC), Food and Agriculture Organization (FAO), and the World Bank. (Go back)
3. The 1992 World Atlas of Desertification (WAD) and the IPCC use the Penman–Monteith method, while the 2018 WAD implements the Thornthwaite method (Cherlet et al., 2018). (Go back)
References
Cherlet, M., Hutchinson, C., Reynolds, J., Hill, J., Sommer, S., & von Maltitz, G. (Eds.). (2018). World Atlas of Desertification. Publication Office of the European Union. https://doi.org/10.2760/9205
D’Odorico, P., Bhattachan, A., Davis, K., Ravi, S., & Runyan, C. (2013). Global desertification: Drivers and feedbacks. Advances in Water Resources, 51, 326–344. https://doi.org/10.1016/j.advwatres.2012.
01.013
FAO. (2011). Highlands and drylands. (N. Berrahmouni, R. Romeo, D. McGuire, S. Zelaya, D. Maselli, & T. Kohler, Eds.). Food and Agriculture Organization of the United Nations.
FAO. (2019). Trees, forests and land use in drylands: The first global assessment. Food and Agriculture Organization of the United Nations. https://www.fao.org/3/ca7148en/ca7148en.pdf
FAO. (2021). The state of the world’s land and water resources for food and agriculture. Systems at breaking point. Synthesis report. Food and Agriculture Organization of the United Nations. https://doi.org/10.4060/cb7654en
Hewitt, K. (1997). Regions at risk. A geographical introduction to disasters. England. Longman.
Le Houérou, H. N. (1984). Rain use efficiency: A unifying concept in arid-land ecology. Journal of Arid Environments, 7, 213–47.
Lucatello, S., & Huber-Sannwald, E. (2020). Sustainable Development Goals and drylands: Addressing the interconnection. In S. Lucatello, E. Huber-Sannwald, I. Espejel, & N. Martinez-Taguena (Eds.), Stewardship of future drylands and climate change in the Global South (pp. 27–40). Springer.
Maestre, F. T. (2012). Plant species richness and ecosystem multifunctionality in global drylands. Science, 214, 214–218. https://doi.org/10.1126/science.1215442
Maestre, F. T., Benito, B. M., Berdugo, M., Concostrina-Zubiri, L., Delgado-Baquerizo, M., Eldridge, D. J., Guirado, E., Gross, N., Kefi, S., Le Bagousse-Pinguet, Y., Ochoa-Hueso, R., & Soliveres, S. (2021). Biogeography of global drylands. New Phytologist, 231(2), 540–558. https://doi.org/10.1111/nph.17395
Maestre, F. T., Salguero-Gomez, R., & Quero, J. L. (2012). It is getting hotter in here: Determining and projecting the impacts of global environmental change on drylands. Philosophical Transactions of the Royal Society B: Biological Sciences, 367(1606), 3062–3075. https://doi.org/10.1098/rstb.2011.0323
Martinez-Valderrama, J. (2016). Los desiertos y la desertificación. Ediciones Catarata.
Millenium Ecosystem Assessment (MEA). (2005). Drylands. In R. Hassan, R. Scholes, & N. Ash (Eds.), Ecosystems and human well being: Scenarios (pp. 623–662). Island Press.
Mirzabaev, A., Wu, J., Evans, J., Garcia-Oliva, F., Hussein, I. A. G., Iqbal, M. M., Kimutai, J., Knowles, T., Meza, F., Nedjraoui, D., Tena, F., Turkeş, M., Vazquez, R. J., & Weltz, M. (2019). Desertification. In J. M. P. R. Shukla, J. Skea, E. Calvo Buendia, V. Masson-Delmotte, H.-O. Portner, D. C. Roberts, P. Zhai, R. Slade, S. Connors, R. van Diemen, M. Ferrat, E. Haughey, S. Luz, S. Neogi, M. Pathak, J. Petzold, J. Portugal Pereira, P. Vyas, E. Huntley, ... J. Malley (Eds.), Climate change and land: An IPCC special report on climate change, desertification, land degradation, sustainable land management, food security, and greenhouse gas fluxes in terrestrial ecosystems (pp. 249–343). UNEP. https://www.ipcc.ch/srccl/chapter/chapter-3/
Plaza, C., Zaccone, C., Sawicka, K., Mendez, A. M., Tarquis, A., Gasco, G., Heuvelink, G. B., Schuur, E. A. G., & Maestre, F. T. (2018). Soil resources and element stocks in drylands to face global issues. Scientific Reports, 8(1), 1–8. https://doi.org/10.1038/s41598-018-32229-0
Reynolds, J. F., Kemp, P. R., Ogle, K., & Fernandez, R. J. (2004). Modifying the –pulse-reserve– paradigm for deserts of North America: Precipitation pulses, soil water, and plant responses. Oecologia, 141, 194–210. https://doi.org/10.1007/s00442-004-1524-4
UN. (2015, 25 September). General Assembly Resolution 70/1, Transforming Our World: The 2030 Agenda for Sustainable Development, A/RES/70/1. http://undocs.org/A/RES/70/1
UNCCD. (2017). The global land outlook (1st ed.). United Nations Convention to Combat Desertification.
UNEP-WCMC. (2007). A spatial analysis approach to the global delineation of dryland areas of relevance to the CBD Programme of Work on Dry and Subhumid Lands. Dataset based on spatial analysis between WWF terrestrial ecoregions (WWF-US, 2004) and aridity zones (CRU/UEA; UNEPGRID, 1991). Dataset checked and refined to remove many gaps, overlaps and slivers (July 2014).
Vanham, D., Alfieri, L., Florke, M., Grimaldi, S., Lorini, V., Roo, A. De, & Feyen, L. (2021). The number of people exposed to water stress in relation to how much water is reserved for the environment: A global modelling study. Lancet Planet Health, 5, 766–774. https://doi.org/10.1016/S2542-5196(21)00234-5