Climate change in high-mountain regions
An international perspective and a look at the Pyrenees
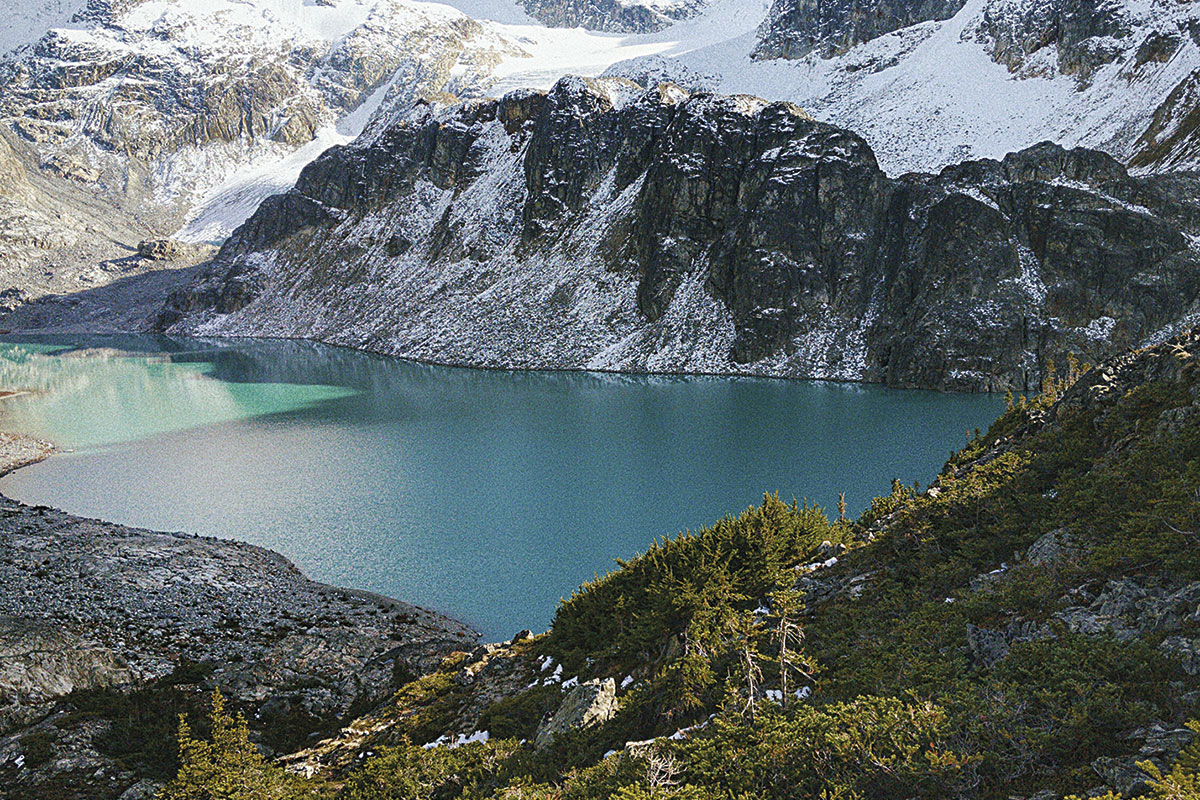
High mountains are among the regions most affected by climate change. The complex network of interactions between climate, biological, and sociocultural structures in these regions is being altered by the changing climate. In this work, we try to explore the future challenges for these unique regions. We analyse why they are important and what problems they are facing in today’s climate and political scenario, with a special focus on the Pyrenees.
Keywords: high mountain, elevation-dependent warming, climate change, cryosphere, biodiversity hotspots, early warning systems.
Introduction
We often imagine mountains as remote, wild, and untouched places, the last corners of our planet not impacted by human activities. But the reality is vastly different: high mountains are suffering the impact of climate change in a particularly intense way. Among other factors, the complex network of interactions between high-mountain habitats and the species they harbour is being altered by the effects of climate change. In this text, we will focus on the challenges facing these unique regions today.
What are high mountains?
It is not easy to find an exact definition of high-mountain areas. The main reason is that the requirement for them to be considered «high» depends on multiple factors that are not exclusively related to elevation. We can find a wide variety of definitions in the scientific literature, depending on the approach and goals of each study. In any case, an adequate definition must identify high-mountain areas as unique environments that are differentiated from the lower topographical elements surrounding them.
In this text, we define high mountains as geological structures in which cryospheric elements such as snow, permafrost, and glaciers play a leading role. Similarly, additional features, especially regarding extreme weather and terrain complexity are also required. We must also consider the importance of spatial and institutional remoteness. These characteristics lead us to perceive high mountains as remote, alien spaces, detached from our lives and societies. But nothing could be further from the truth: mountains are the source and refuge of resources and goods that are essential in our everyday lives. In this sense, these regions are unquestionably important from an ecological, social, and economic point of view (Figure 1).
Water towers of the planet | Biodiversity hotspots | Traditional and ancestral knowledge |
Cryosphere characteristics
The presence of glaciers, permafrost, and snow is a major feature of high-mountain regions. These elements also play vital roles in the planet’s hydrological cycle. So much so that they contribute to the seasonal and long-term storage of water resources for roughly half of humanity. Hence, they are considered the planet’s «water towers» (Viviroli et al., 2007). |
Ecosystem characteristics
In tropical and subtropical high-mountain regions, a wide variety of ecosystems coexist in a small area, ranging from tropical to polar conditions. This produces endemisms that are particular to high mountains and is one of the factors responsible for the high biodiversity. The geological dynamics of mountain creation interacts with complex climate changes to provide an unparalleled opportunity for specific evolutionary processes to unfold (Rahbek et al., 2019). |
Social characteristics
High-mountain social structures are also a key characteristic. It is estimated that in 2010, a population of around 670 million people, representing 10 % of the world’s population, was living in these regions. Apart from providing us with material resources such as water and food, high-mountain regions are also home to the unique traditional and ancestral heritage of indigenous and traditional communities. |
The issue
The geographic and environmental conditions described above mean that high mountains are valuable and unique regions, thereby making them some of the territories most vulnerable to climate change. Their abrupt relief and steep slopes hide an intrinsic fragility. This vulnerability is related to a faster and more intense response to changes than non-mountainous regions (Diaz et al., 2003), and is understood by many experts as a potential early warning system. In other words, the impacts we are starting to observe in high mountains today might be warning us of the worst consequences we may suffer in the not-too-distant future.
Climate change
High-mountain regions are (and will continue to be) among those most affected by climate change. According to the conclusions of the Special Report on the Ocean and Cryosphere in a Changing Climate (SROCC; Intergovernmental Panel on Climate Change [IPCC], 2019), the increase in surface air temperature predicted in 21st century climate models may be more intense in high mountains because of regional dynamics and an effect called elevation-dependent warming (EDW). This process refers to the observation that the rate of warming (expressed in °C per decade, for instance) is not the same for all elevation ranges (Pepin et al., 2015). The increase in temperature directly impacts the rainfall/snowfall rates in high-mountain areas. Snowfall is projected to dwindle in most mountain areas, especially at lower elevations. The IPCC (2019) considers it probable that, by the end of the century (2081–2100), the snow cover at lower elevations in regions such as the European Alps, Himalayas, and Subtropical Andes will be reduced by 30 % in the best-case scenario and 80 % in the worst, compared to our near past (1986–2005).
The mass loss of glaciers is also accelerating (Hugonnet et al., 2021). Although the loss in glacier mass greatly varies between regions, best-case and worst-case scenario projections (depending on the concentration of CO2 in the atmosphere) indicate that globally, polar and mountain glaciers will lose between 18 % and 36 % of their masses, respectively, during the 21st century, compared to 2015 (IPCC, 2019). This loss of ice and snow in high-mountain areas stems from the increase in the Earth’s average temperature due to the constant anthropogenic emission of greenhouse effect gases since the Industrial Revolution.
Several examples of these changes have been documented. For example, in the Cordillera Blanca (Peruvian Andes), more than 30 % of the glacier mass disappeared between 1930 and 2014 (Schauwecker et al., 2014). Another illustrative example is that of Mount Kilimanjaro, whose glacier area suffered a severe loss of 85 % between 1912 and 2011 (Cullen et al., 2013). However, looking for such remote places is not necessary to observe the effects of climate change. In regions dominated by smaller glaciers, including the European Alps, Caucasus, and Pyrenees, among others, glaciers are predicted to lose more than 80 % of their masses (IPCC, 2019) by 2100, according to the IPCC’s worst-case scenario (RCP8.5). Indeed, many of the glaciers in these mountain ranges will completely disappear in the future with the current increase in global temperature.
These cryospheric changes caused by climate change generate biophysical impacts such as the loss of stationary water, which directly affects ecosystems and has socio-economic impacts on the inhabitants of these regions (Figure 2). Biophysical impacts materialise in a wide variety of forms: from landslides and avalanches to the disappearance of species. Glacial lake outburst floods (GLOFs) are some of the most dangerous of these phenomena. These are defined as violent floods occurring when the containment elements of a glacial lake (i.e., glacier ice) collapse. In 2010, more than 200 lakes were identified as potentially affected by this danger in the Hindu-Kush–Himalaya alone (Ives et al., 2010). Another hazard derived from these climate changes is the transformation of areas that were already sensitive to wildfires, such as California’s coastal mountain ranges or the Blue Mountains in Australia, regions facing a continued risk of wildfires, or the transformation of areas such as Tibet or Mongolia into regions prone to desertification (IPCC, 2019).
Figure 2. Climate changes in high-mountain areas and their associated biophysical and socio-economic impacts. / Source: Based on Huggel et al. (2019)
In addition to some of the biophysical impacts mentioned above, climate changes produce significant imbalances in the ecological, social, and economic structures of high-mountain areas, which can sometimes result in the loss of human lives. A clear example of this are fatalities related to avalanches and landslides or droughts and flooding. Although some initiatives to help adaptation to these new circumstances are already under way, many communities will not be able to adapt, which could lead to conflicts related to access to water and other resources. In fact, economic losses associated with these impacts (related to hydroelectric production and climate disasters) amount to billions of dollars. Indeed, between 1985 and 2014, the economic losses derived from hydrometeorological disasters are estimated to have amounted to 45 billion dollars in the Hindu-Kush–Himalaya and 7 billion dollars in the European Alps region (Stäubli et al., 2018).
However, beyond the economic cost, together with high-mountain ecosystems, we would also lose heritage that belongs to all of us. Traditional mountain communities are already experiencing changes in their livelihoods (e.g., shepherding and agriculture) associated with changes in the water supply. In remote high-mountain areas, indigenous communities are losing both their property and their cultural identity. For instance, in the vicinity of the Ausangate Mountain in Peru, the Quechua community has stopped celebrating traditional rites related to the deity of a now-disappeared glacier. The landscape is also being altered. It is intricately connected not only to cultural identity, but also to the economy of mountain societies, because tourism often represents the most important economic activity in these regions (Palomo, 2017).
The great unknown
Despite evidence of the harmful (sometimes even catastrophic) effects of climate change on high-mountain areas, the current lack of knowledge of these dynamics can negatively affect the adoption of measures aimed at alleviating their effects. This is because, in the context of climate change, mountains continue to be poorly understood.
Two of the main reasons for our ignorance are the inaccessibility of the terrain and the fragmentation of stakeholders, which is evident, for example, in the lack of cross-border initiatives. Observational data are often scarce, too recent, or sometimes just low quality. This means that our current information is biased and inaccurate and tends to be useless when trying to correctly capture small scale changes (IPCC, 2019). However, it is important to highlight that there are specific cases with different realities in many mountain areas. In mountain ranges from rich and developed regions with a long history of mountain research, more and better climate data are available which cover a sufficient timescale to allow us to conduct robust climate studies.
Future changes are also predicted by global and regional climate models which are still incapable of precisely reproducing the details of meso- and sub-kilometre-scale dynamics in complex terrain areas. Mesoscale convective systems, for example, play a critical role in mountain areas and control weather variables such as rainfall (Gutowski et al., 2020). Thanks to advances in computational techniques and the efforts of the scientific community, great progress is already being made towards the incorporation of these processes into models. However, especially in understudied regions, the tools currently available are sometimes insufficient when it comes to comprehensively evaluating the impacts of climate change.
Another complication for the research of high-mountain areas in the context of climate change is the fact that, traditionally, efforts have focused on understanding the dynamics of each mountain range separately and independently. This is because each region will be affected in diverse ways depending on characteristics such as geographical location, area, elevation, and climate pattern. However, these unique regions also share common elements that are key to understanding them globally. Thus, the scientific community has focused on studying not only the singularities of each high-mountain region but are also grouping them together as closely related regions with common characteristics. This comprehensive approach implies a transdisciplinary perspective that allows us to jointly analyse the causes and effects of climate change on high-mountain regions.
The forgotten
Although some mountain regions have been assigned great importance in particular local and regional contexts for decades, the situation is different at the global level. In 2015, the foundations were set for an innovative global action plan for a combined response to the problems faced in high-mountain regions. Three key programmes were adopted that year: the Paris Agreement, which specifically mentions the protection of early warning areas, the United Nations Sustainable Development Agenda with its Sustainable Development Goals (SDGs), and the Sendai Framework for disaster risk reduction.
However, even though the foundations are there, according to the SROCC report (IPCC, 2019), experts state that there is little evidence available to allow us to systematically evaluate the effectiveness of international programmes when addressing specific challenges related to changes in high-mountain ecosystems and their cryosphere.
Thus, to prevent high mountains in the most remote regions from falling into «oblivion» in terms of international policy, we must redirect the approach of these programmes to focus on the context of mountain regions (Bracher et al., 2018). In this sense, some initiatives such as defining specific key considerations to improve the conditions under which the SDGs can have a purpose in mountain regions, are already being developed.
A special look at the Pyrenees
The three global threats – climate change, ignorance, and neglect – do not apply to every mountain region in the world in the same way. In the specific case of the Pyrenees, the effects of climate change have been noted over the last century. Indeed, in this region, the average temperature has increased markedly over the last 50 years. In addition, precipitation is decreasing by 2.5 % per decade (Amblar-Francés et al., 2020). According to climate model estimates, the annual maximum temperature in the Pyrenees for the 2030 horizon will increase by 1.0 to 2.7 °C compared to 1961–1990 levels in the RCP8.5 scenario (Amblar-Francés et al., 2020). Furthermore, by 2050, warming would be higher, ranging from 2.0 to 4.0 °C (Amblar-Francés et al., 2020). Glaciers are also at risk. More specifically, over the last 150 years, the Monte Perdido Glacier (Huesca, Spanish Pyrenees) has experienced more pronounced melting than in the last 2,000 years and data suggests that, under these climatic conditions, it will eventually disappear (Moreno et al., 2021).
Because of these climate changes, many species’ annual life cycles are starting earlier, thus affecting the interactions between different species (Charmantier & Gienapp, 2014). Lakes and peatlands, iconic ecosystems of the Pyrenees, are also at risk of disappearing because of their particular vulnerability (Catalan et al., 2006).
«Biophysical impacts materialise in a wide variety of forms: from landslides and avalanches to the disappearance of species»
One of the most critical factors in this region is water availability. Among the many contributions of the Pyrenees, one of the most important is the fundamental function of supplying water to the surrounding territories. This is because a large part of the surface and underground affluents that feed the basins of the rivers Ebro, Bidasoa, Adour, Garonne, and Aude, among others, originate in this mountain range. More specifically, the Pyrenees account for 70 % of the total inflow to the river Ebro. Therefore, these mountains are a key element in the supply of water not only for agriculture and electricity production, but also for industry and domestic consumption. The combined effect of the change in climate and land use will significantly alter the patterns and quality of water resources both in the Pyrenees and in a much wider territory that affects millions of inhabitants. This effect will have a special impact on the low-lying northern regions of the peninsular Mediterranean slope, which are major water consumers, including water-scarce and densely populated coastal regions (Observatorio Pirenaico para el Cambio Climático – Comunidad de Trabajo de los Pirineos [OPCC-CTP], 2018).
The Pyrenees are one of the great mountain ranges in Europe and one of the ranges with the highest density of stations for measuring environmental variables in the world. Compared to other high-mountain ranges in the world, the location of the region is more favourable for monitoring the effects of climate change and the time scale of these effects. Furthermore, this mountain range features several agents that actively participate in the process of monitoring, researching, adapting, mitigating, and communicating climate change, including the IPE-CSIC (Pyrenean Institute of Ecology) and the CBNPMP (Conservatoire Botanique National des Pyrénées et de Midi-Pyrénées). In addition, the cross-border initiative (Spain–Andorra–France) of the Pyrenean Climate Change Observatory (OPCC), which launched in 2010, aims to promote territorial collaboration on climate change.
However, it was not until 2020 that a high-resolution study of climate evolution was conducted in this mountain range (Amblar-Francés et al., 2020). Nonetheless, although we are on the right track, there is a lot to be done in terms of generating scientific knowledge that integrates the entire mountain range into the assessment of biophysical and socio-cultural impacts on the Pyrenees and the surrounding territories (OPCC-CTP, 2018).
Conclusions
The scientific community is warning us of the losses that these ecosystems will suffer. It is therefore vitally important to consolidate quality hydrological, meteorological, and climatic services that are, in turn, tailored to the specific risks and needs of mountain areas. Similarly, international cooperation-for-development policies must be reviewed and updated to incorporate and integrate policies for the sustainable development and conservation of mountain ecosystems. At the regional and local level, we must promote initiatives capable of strengthening the relationship between political decisions, scientific results, and traditional and indigenous knowledge. These solutions must be based on collaboration with stakeholders, actively including them in the decision-making process.
In more privileged regions, these actions have been in place for decades, but there is a strong contrast with poorer and more remote mountain regions. Thus, it will be essential to translate this progress and homogenise the quality and quantity of mountain climate information. In the context of providing novel solutions, the World Meteorological Organization held the High Mountain Summit in October 2019. It was attended by many major international entities, including the World Bank Group (WBG), the UN’s FAO (Food and Agriculture Organization), and UNESCO (the United Nations Educational, Scientific and Cultural Organization). The result was an unprecedented call to action addressed to all social sectors, including governments, scientists, and academics, as well as private and civil society entities (World Meteorological Organization [WMO], 2019).
Real change, however, depends on making society as a whole aware of these problems, which is why institutions at all levels, from local to international, must promote activities to inform and raise public awareness about the incalculable value of these regions and the serious impacts they are suffering and will suffer in the years to come as a result of climate change. The challenge facing high-mountain areas is real, and so too must be the actions implemented to address it.
References
Amblar-Francés, P., Ramos-Calzado, P., Sanchis-Lladó, J., Hernanz-Lázaro, A., Peral-García, M. C., Navascués, B., Dominguez-Alonso, M., Pastor-Saavedra, M. A., & Rodríguez-Camino, E. (2020). High resolution climate change projections for the Pyrenees region. Advances in Science and Research, 17, 191–208. https://doi.org/10.5194/asr-17-191-2020
Bracher, C. P., von Dach, S. W., & Adler, C. (2018). Challenges and opportunities in assessing sustainable mountain development using the UN Sustainable Development Goals. CDE Working Paper, 3. Centre for Development and Environment (CDE). http://doi.org/10.7892/boris.119737
Catalan, J., Camarero, L., Felip, M., Pla, S., Ventura, M., Buchaca, T., Bartumeus, F., de Mendoza, G., Miró, A., Casamayor, E. O., Medina-Sánchez, J. M., Bacardit, M., Altuna, M., Bartrons, M., & Díaz de Quijano, D. (2006). High mountain lakes: Extreme habitats and witnesses of environmental changes. Limnética, 25, 551–584. http://hdl.handle.net/10261/44375
Charmantier, A., & Gienapp, P. (2014). Climate change and timing of avian breeding and migration: Evolutionary versus plastic changes. Evolutionary Applications, 7(1), 15–28. https://doi.org/10.1111/eva.12126
Cullen, N. J., Sirguey, P., Mölg, T., Kaser, G., Winkler, M., & Fitzsimons, S. J. (2013). A century of ice retreat on Kilimanjaro: The mapping reloaded. The Cryosphere, 7, 419–431. https://doi.org/10.5194/tc-7-419-2013
Diaz, H. F., Grosjean, M., & Graumlich, L. (2003). Climate variability and change in high elevation regions: Past, present and future. Advances in Global Change Research. Springer. https://doi.org/10.1007/978-94-015-1252-7
Gutowski, W. J., Ullrich, P. A., Hall, A., Leung, L. R., O’Brien T. A., Patricola, C. M., Arritt, R. W., Bukovsky, M. S., Calvin, K. V., Feng, Z., Jones, A. D., Kooperman, G. J., Monier, E., Pritchard, M. S., Pryor, S. C., Qian, Y., Rhoades, A. M., Roberts, A. F., Sakaguchi, K., … Zarzycki, C. (2020). The ongoing need for high-resolution regional climate models: Process understanding and stakeholder information. Bulletin of the American Meteorological Society, 101(5), E664–E683. https://doi.org/10.1175/BAMS-D-19-0113.1
Huggel, C., Muccione, V., Carey, M., James, R., Jurt, C., & Mechler, R. (2019). Loss and damage in the mountain cryosphere. Regional Environmental Change, 19(5), 1387–1399. https://doi.org/10.1007/s10113-018-1385-8
Hugonnet, R., McNabb, R., Berthier, E., Menounos, B., Nuth, C., Girod, L., Farinotti, D., Huss, M., Dussaillant, I., Brun, F., & Kääb, A. (2021). Accelerated global glacier mass loss in the early twenty-first century. Nature, 592(7856), 726–731. https://doi.org/10.1038/s41586-021-03436-z
IPCC. (2019). IPCC special report on the ocean and cryosphere in a changing climate. H.-O. Pörtner, D. C. Roberts, V. Masson-Delmotte, P. Zhai, M. Tignor, E. Poloczanska, K. Mintenbeck, A. Alegría, M. Nicolai, A. Okem, J. Petzold, B. Rama, N. M. Weyer (Eds.). In press.
Ives, J., Shrestha, R., & Mool, P. (2010). Formation of glacial lakes in the Hindu Kush-Himalayas and GLOF risk assessment. ICIMOD. https://www.preventionweb.net/files/14048_ICIMODGLOF.pdf
Moreno, A., Bartolomé, M., López-Moreno, J. I., Pey, J., Corella, J. P., García-Orellana, J., Sancho, C., Leunda, M., Gil-Romera, G., González-Sampériz, P., Pérez-Mejías, C., Navarro, F., Otero-García, J., Lapazaran, J., Alonso-González, E., Cid, C., López-Martínez, J., Oliva-Urcia, B., Faria, S. H., … García-Ruíz, J. M. (2021). The case of a southern European glacier disappearing under recent warming that survived Roman and Medieval warm Periods. The Cryosphere, 15, 1157–1172. https://doi.org/10.5194/tc-15-1157-2021
Observatorio Pirenaico para el Cambio Climático – Comunidad de Trabajo de los Pirineos [OPCC-CTP]. (2018). El cambio climático en los Pirineos: Impactos, vulnerabilidades y adaptación. OPCC-CTP.
Palomo, I. (2017). Climate change impacts on ecosystem services in high mountain areas: A literature review. Mountain Research and Development, 37(2), 179–187. https://doi.org/10.1659/MRD-JOURNAL-D-16-00110.1
Pepin, N., Bradley, R. S., Diaz, H. F., Baraer, M., Cáceres, E. B., Forsythe, N., Fowler, H., Greenwood, G., Hashmi, M. Z., Liu, X. D., Miller, J. R., Ning, L., Ohmura, A., Palazzi, E., Rangwala, I., Schöner, W., Severskiy, I., Shahgedanova, M., Wang, M. B., …Yang, D. Q. (2015). Elevation-dependent warming in mountain regions of the world. Nature Climate Change, 5(5), 424–430. https://doi.org/10.1038/nclimate2563
Rahbek, C., Borregaard, M. K., Antonelli, A., Colwell, R. K., Holt, B. G., Nogues-Bravo, D., Rasmussen, C. M. Ø., Richardson, K., Rosing, M. T., Whittaker, R. J., & Fjeldså, J. (2019). Building mountain biodiversity: Geological and evolutionary processes. Science, 365(6458), 1114–1119. https://doi.org/10.1126/science.aax0151
Schauwecker, S., Rohrer, M., Acuña, D., Cochachin, A., Dávila, L., Frey, H., Giráldez, C., Gómez, J., Huggel, C., Jacques-Coper, M., Loarte, E., Salzmann, N., & Vuille, M. (2014). Climate trends and glacier retreat in the Cordillera Blanca, Peru, revisited. Global Planetary Change, 119, 85–97. https://doi.org/10.1016/j.gloplacha.2014.05.005
Stäubli, A., Nussbaumer, S., Allen, S. K., Huggel, C., Arguello, M., Costa, F., Hergarten, C., Martínez, R., Soto, J., Vargas, R., Zambrano, E., & Zimmermann, M. (2018). Analysis of weather- and climate-related disasters in mountain regions using different disaster databases. In S. Mal, R. B. Singh, & C. Huggel (Eds.), Climate change, extreme events and disaster risk reduction (pp. 17–41). Sustainable Development Goals Series. Springer. https://doi.org/10.1007/978-3-319-56469-2_2
Viviroli, D., Dürr, H, H., Messerli, B., Meybeck, M., & Weingartner, R. (2007). Mountains of the world, water towers for humanity: Typology, mapping, and global significance. Water Resources Research, 43(7), W07447. https://doi.org/10.1029/2006WR005653
World Meteorological Organization [WMO]. (2019). High Mountain Summit. Retrieved February 1, 2021, from https://highmountainsummit.wmo.int/en